Efforts to research and develop quantum computing have advanced far enough in the past decade to allow us to separate hype from reality and truly understand where quantum computing will have the biggest impact. At Microsoft, we believe that it will take the world’s collective genius to realize the full potential of quantum computing. Our goal is to both empower and help focus that genius in areas that show the greatest opportunity. To that end, Microsoft collaborated with Torsten Höfler, a leader in high-performance computing on new guidance for quantum application development that was just published: Disentangling Hype from Practicality: On Realistically Achieving Quantum Advantage.
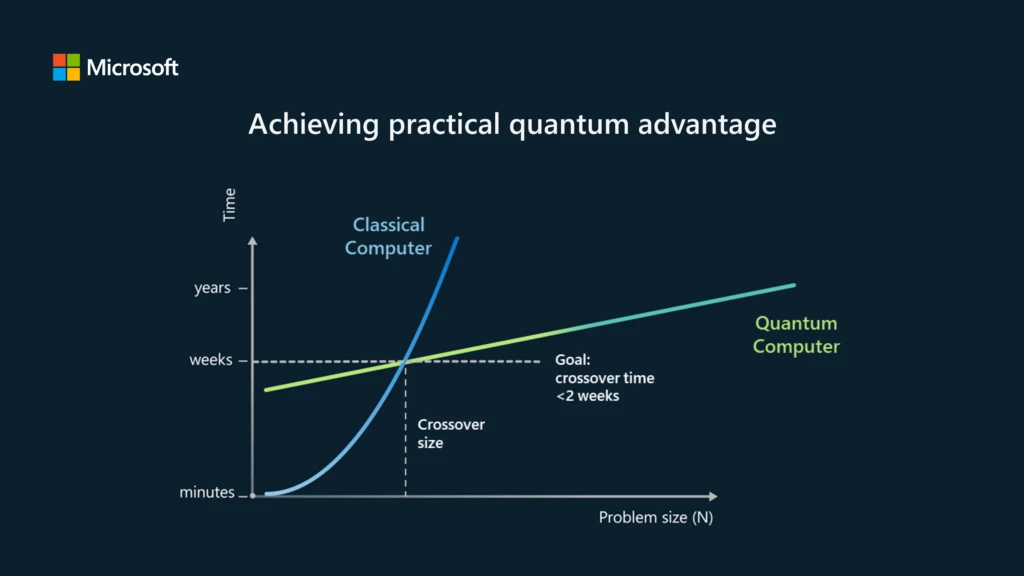
The promise of quantum computing at scale is real. It will solve some of the hardest challenges facing humanity. However, it will not solve every challenge. There is an ever-growing list of applications being explored for quantum computing today ranging from logistics, cosmology and financial market prediction to carbon capture, big data analysis, biochemistry, and many more. It’s clear that business, academic, and government leaders are turning to our industry with great hope. However, such optimism needs to be measured. The areas where quantum will have its biggest impact are coming more clearly into focus. The fundamentals of quantum physics govern which problems can benefit from the capabilities of quantum systems. Specifically, finding algorithms that present a significant advantage for quantum systems to justify their additional cost is the first hurdle. In addition, problems cannot rely on massive quantities of data, as getting data into and out of quantum systems presents a major bottleneck.
Quantum computers have different characteristics than classical systems, and so are useful for a different set of problems. Creating a practical quantum advantage, or quantum practicality, is relatively rare because of the specific characteristics that make a quantum computer effective.
A framework for quantum practicality
How can we determine which problems will benefit most from quantum computers?
To find out, we created a general model of quantum computer capabilities and shortcomings. We assume that researchers will be able to create a scaled quantum computer and compared its hypothetical performance to one of today’s classical computers with a single state-of-the-art GPU. We assume the scaled quantum computer will have 10,000 fast, error-corrected logical qubits, or about one million physical qubits (a machine like the one Microsoft is engineering right now).
A major benefit of quantum systems is that they use the quantum foundations of nature, which results in much greater efficiency, and thus fewer operations, when applied to a specific subset of problems compared to a classical computer. However, as quantum computers take a longer time for each operation, a quantum algorithm must offer significant speedups to overcome the larger complexity of each operation. But what does that mean concretely?
For our analysis, we set a break-even point of two weeks, meaning a quantum computer should be able to perform better than a classical computer on problems that would take a quantum computer not more than two weeks to solve. Comparing the hypothetical future quantum computer to a single classical GPU available today, one finds that more than quadratic speedup—and ideally super-polynomial speedup—is needed. This is a significant finding since many proposed applications of quantum computing rely on the quadratic speedup of specific algorithms, such as Grover’s algorithm.
In addition, again due to the larger complexity of each operation, quantum computers have limited bandwidth to run operations. In fact, even a scaled quantum computer will only be able to handle 1/10,000th of the bandwidth of specialized computer processors, such as the graphics processing units often used for machine learning. Because of the limited data sizes that quantum computers can process, this “quantum speedup” must happen with relatively simple forms of the problem of interest.
This combination of specific algorithms that exhibit a great enough quantum speedup and problems that can be expressed with a limited amount of data determines the boundaries of what current quantum systems are capable of and what scaled systems might achieve in the future. Applying these optimistic assumptions for quantum computers to different application domains gives us a way to determine where quantum computers will have the greatest impact in the future.
Computational chemistry and materials science will benefit tremendously from scaled quantum computing
One of the ideal applications for quantum computing is in simulating chemical interactions and materials on the quantum level.
Many problems facing the world today boil down to chemistry and material science problems. Better and more efficient electric vehicles rely on finding better battery chemistries. More effective and targeted cancer drugs rely on computational biochemistry. And materials that can last long enough to be useful, but then biodegrade quickly afterwards, rely on discoveries in these fields.
If quantum computers only benefited chemistry and material science, that would be enough. There is a reason that the major eras of innovation—Stone Age, Bronze Age, Iron Age, Silicon Age—are named for materials. Innovations in chemistry and material science are estimated to have an impact on 96 percent of all manufactured goods, which impact 100 percent of humanity.
Our framework for evaluating quantum practicality shows that chemistry and material science problems benefit from quantum speedup because activities like simulating the interactions of a single chemical can be represented by a limited number of interaction strengths between electrons in their orbitals. While many approximate calculations of their properties are routinely performed, the operations are exponentially complex on classical computers, but efficient on quantum computers, falling within our stated guidelines.
While scaled quantum computing is required to solve the hardest, most complex chemistry and materials science problems, progress can be made today with Azure high-performance computing. For example, Johnson Matthey and Microsoft Azure Quantum chemists have accelerated some quantum chemistry calculations in their search for hydrogen fuel cell catalysts by combining high-performance computing and specific quantum functions to reduce the turnaround time for their scaled workloads from six months to a week.
Where to focus innovation
Our research revealed that applications that rely on large datasets are better served by classical computing, because the bandwidth is too low on quantum systems to allow for applications such as searching databases or training machine-learning models on large datasets. These include applications such as drug design approaches and protein folding that rely on Grover’s algorithm as well as other simulations, such as weather and climate prediction, that rely on large systems of equations.
The limits of getting data into and out of quantum computers will limit the applicability of the systems to more compact problems. Anybody focused on building applications like these will most likely come to find the best results with Azure HPC and AI services. For quantum innovators who are focused on researching new quantum applications beyond chemical and materials science, we are eager to learn what you discover. To support your exploration, we provide the tools, like our Resource Estimator, as well as the skills and state-of-the-art hardware with Azure Quantum.
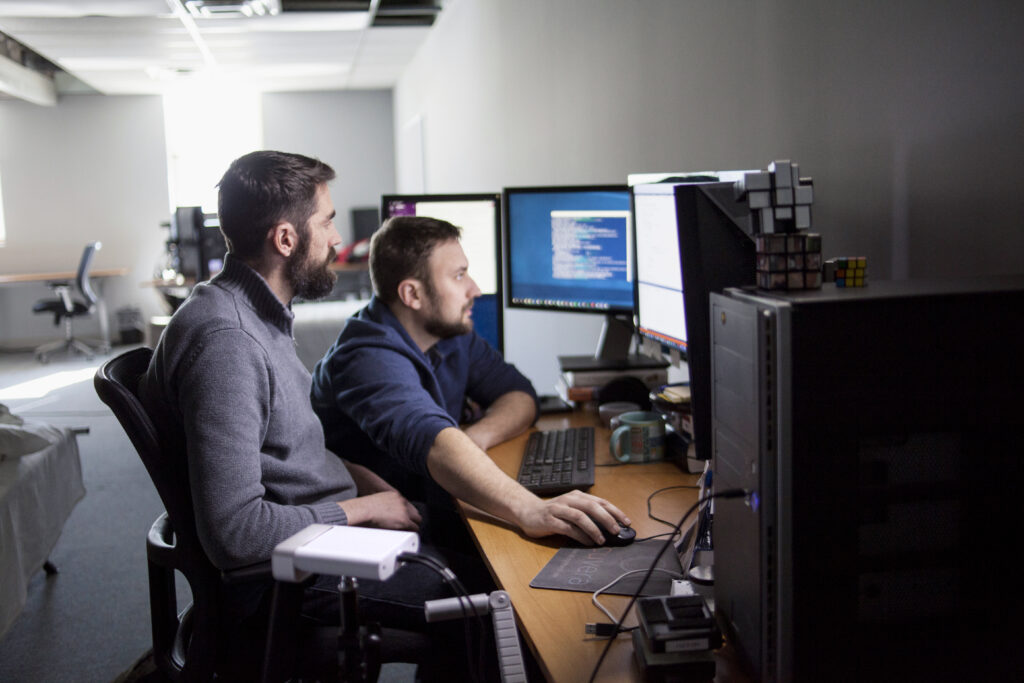
I’m motivated to see the benefits of quantum realized in my lifetime—it is a gift I want to give my kids and their kids. That desire animates my life’s work. To make real progress though, as I said earlier, it will take our collective genius. The more focus scientists can apply to the areas that show the most promise, the sooner we’ll be able to reap the benefits of quantum at scale. Therefore, I’m calling on this community to double down on learning about and researching applications related to computational chemistry and material science.
Accelerate scientific discovery with Azure Quantum
We invite anyone interested in accelerating their chemistry and materials science R&D to join us. To learn more, please check out the latest edition of the Microsoft Quantum Innovator Series: How can we solve quantum problems today and in the future?
If you are interested in meeting with our chemists and quantum architects to learn how our platform can accelerate your R&D, please reach out to the Azure Quantum team at QuantumInnovation@microsoft.com.